Semester and Master's Projects
We offer a wide spectrum of projects for the semester and diploma work in the experimental solid state physics. Some of these projects are carried out in our laboratory at the ETH Honggerberg campus. Others involve experiments at large scale neutron scattering facilities available worldwide and at Paul Scherrer Institute (30 km from ETH).
Since our program is a very active one, the list on this page is most certainly not complete. Please do not hesitate to contact Prof. Dr. A. Zheludev for the latest information.

Published 29/01/2025
Quantum antiferromagnets on a triangular lattice pose one of the oldest and most influential paradigms in quantum magnetism [1]. The highly frustrated lattice geometry tends to inhibit classical long-range order and instead promotes exotic quantum phases of matter. While the simple S=1/2 Heisenberg system has been studied extensively, even a modest exchange anisotropy may dramatically affect the physics and lead to novel ground states or excitations [2], many of which have yet to be realized in experiment.
We have recently synthesized a new family of quantum antiferromagnets on the triangular lattice, which seems to combine a small spatial anisotropy in the triangular plane with the spin-space anisotropies inherent to heavy 4d/5d transition metals with strong spin-orbit coupling. In this Master project, you will learn state-of-the-art techniques of performing calorimetric and magnetic measurements at ultra-low temperatures (down to 50mK) and high magnetic fields (up to 14 T). You will investigate the thermodynamics of one of these new quantum materials, allowing you to map out its magnetic phase diagram. This should give us a solid basis for understanding the complex magnetic behaviour of these materials, likely contributing to a high-profile journal publication.
[1] external page L. Balents, Nature 464, 199–208 (2010)
[2] external page O Starykh, Rep. Prog. Phys. 78 05202 (2015).
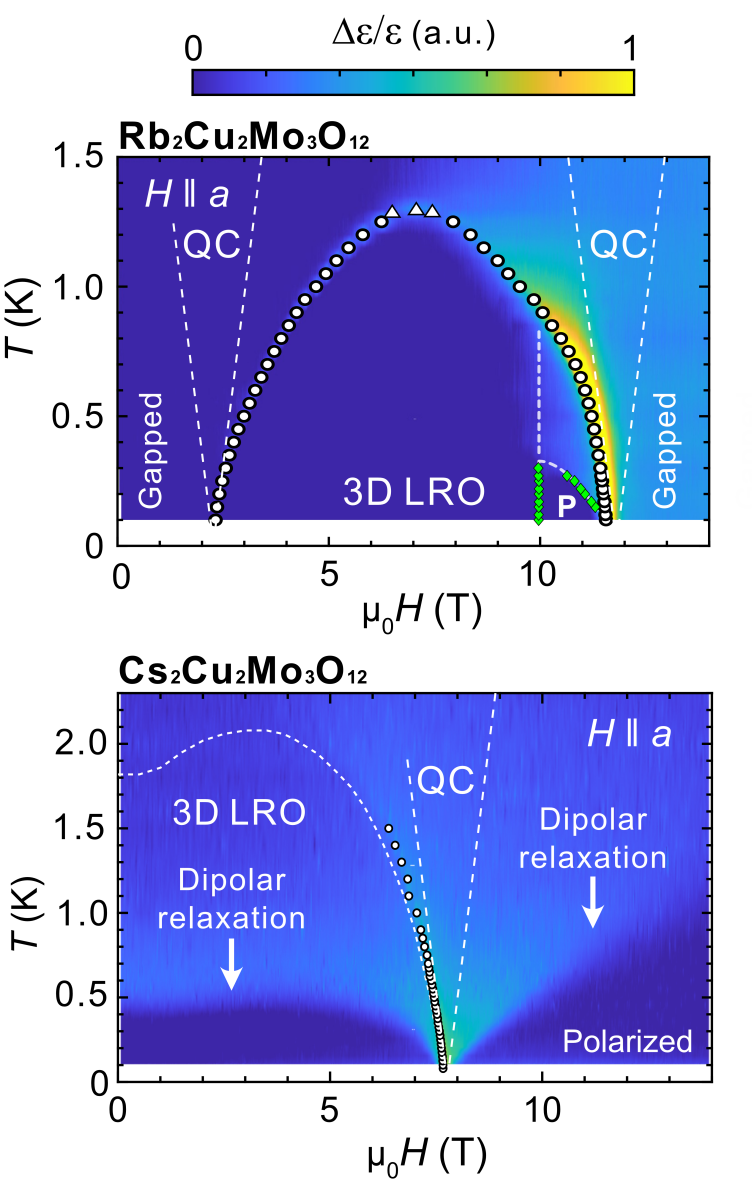
Published: 03/07/2023
Contact: or
The ferro-antiferromagnetic spin chains A2Cu2(MoO3)4 (with A = Rb or Cs) show extremely rich physics due to frustration. In addition to complex magnetism, a strong magnetoelectric coupling opens the way to a variety of exotic magnetoelectric phenomena. Both systems display magnetic Bose-Einstein condensations of magnons, where usually inaccessible critical exponents have been measured [1]. However, despite the structural similarities, measurements of the dielectric permittivity show qualitative differences between them. Cs2Cu2Mo3O12 shows modulations of permittivity with a strong magnetic-field dependence both in the long-range ordered phase and in the paramagnetic phase. This effect has been recently interpreted as a newly-observed relaxation of low-energy electric dipoles mediated by critical magnons [2]. This effect is absent in Rb2Cu2Mo3O12 and its origin is still unclear.
In this Master's Project, you will be part of a detailed study of the structure of these two magnetic systems. You will take part in a series of Synchrotron experiments in large-scale facilities such as ESRF (Grenoble, France) or Diamond (Oxfordshire, UK). Using a combination of single-crystal and polycrystal techniques, the structure of this family of magnets will be thoroughly investigated in the search for an elusive lattice symmetry reduction. These experiments will clarify the different magnetic behavior of the compounds, and pinpoint the microscopic origin of the novel coupling mechanism found in Cs2Cu2Mo3O12. This project will most likely contribute to a publication in a high-impact journal.
References:
[1] external page S. Hayashida, D. Flavian et al., Phys. Rev. Res., 3, 033053 (2021).
[2] external page D. Flavian et al., J. Phys. Rev. Lett., 130, 216501 (2023).
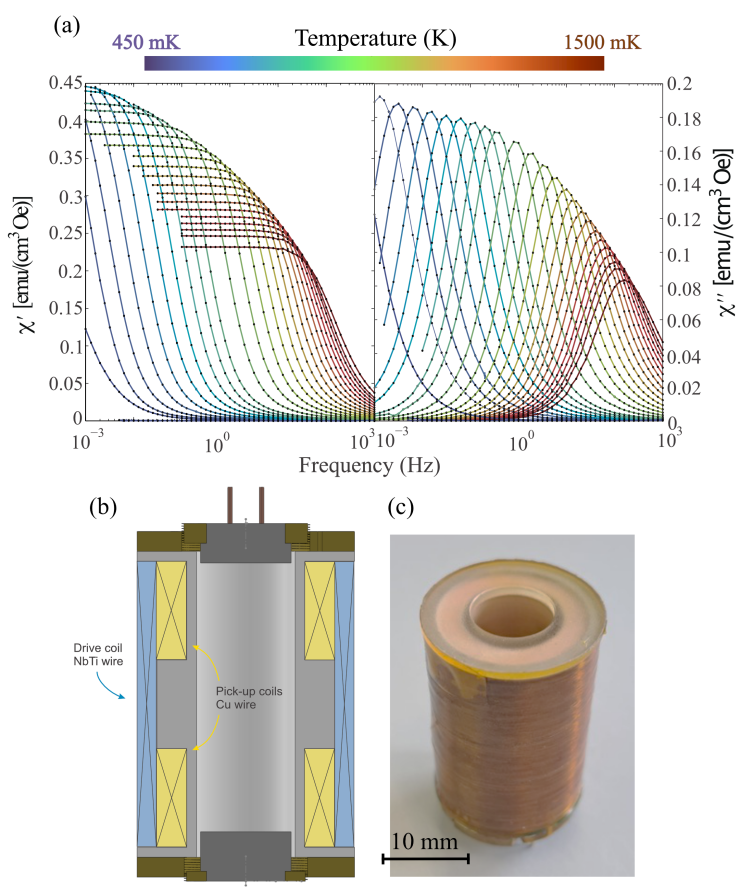
Published: 03/07/2023
Contact: or
Magnetic susceptibility is an essential quantity to study in condensed matter systems. It provides invaluable information on the microscopic properties of materials, ranging from magnetic insulators, to spin glasses and even superconductors. Beyond static bulk properties, AC susceptibility gives also access to dynamical processes, like relaxation mechanisms [1]. As such, it is a widespread technique down to ~ 2 K and many commercial setups are in the market. However, state-of-the-art research in quantum magnetism demands extreme experimental conditions that are at odds with commercial solutions. Extremely versatile low-power, high-throughput devices are required to access the milli-Kelvin regime in the presence of strong magnetic fields (> 10 T). We took up this endeavor and are currently developing our custom platform for AC susceptibility measurements.
In this Master's Project, you will develop a custom-made low-temperature susceptometer. The design will be based on a compact superconducting coil [2], specifically designed in collaboration with industry for this project. Several approaches will be taken to enhance the sensitivity of the device while keeping it low-power. In addition, you will gain insight into several areas of magnetism; various quantum materials are already available and urge for low-temperature AC susceptibility measurements. Finally, your work will contribute to establishing a cutting-edge measurement technique and will likely be part of several publications in high-impact journals.
References:
[1] external page S. Yaraskavitch et al., Phys. Rev. B, 85, 020410(R) (2012).
[2] external page L. Yin, et al.; J. Low Temp. Phys. 158, 710 (2010).
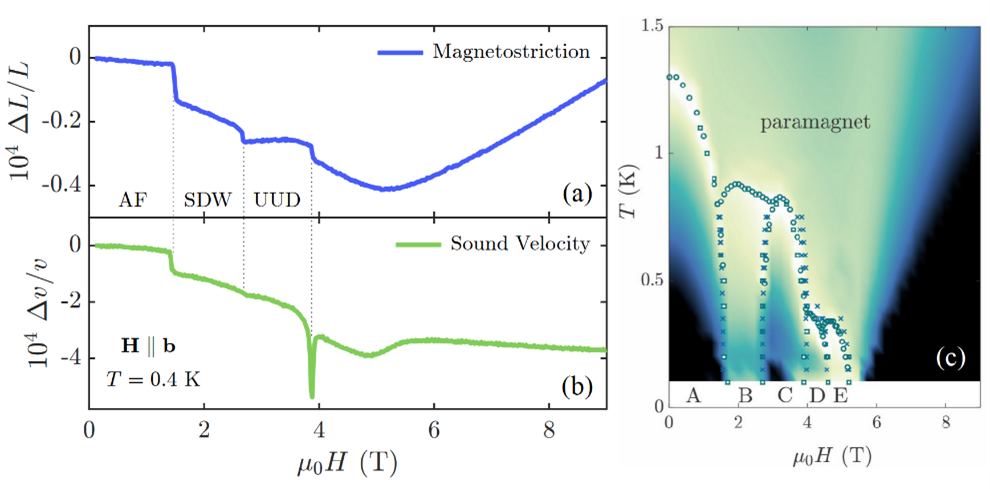
Published 10/01/2023
Contact: or
In magnetically frustrated systems, competing interactions give rise to a plethora of exotic magnetic phases. Even at zero temperature, these can be accessed by tuning an external control parameter like magnetic field or pressure, leading to significant renormalization of thermodynamic properties. Case in point, the magneoelastic coupling – an often neglected interaction between the spin and lattice degrees of freedom – can exhibit anomalous behaviour close to such quantum phase transitions.
The goal of this master thesis shall be an investigation of the magnetoelastic properties in Cs2CoBr4, a novel frustrated quantum material realizing a complex phase diagram with five distinct magnetic phases [1 ,2]. You will complete commissioning of our recently established dilatometric and ultrasonic measurement setups by carrying out systematic high precision measurements at cryogenic temperatures and high magnetic fields. You will determine the magnetostriction – changes in sample length in response to a field – and sound velocity – the speed of propagating lattice vibrations – of Cs2CoBr4 as a function of external parameters. This will elucidate how the lattice is affected by magnetic correlations and vice versa, giving us insights into the complex magnetism of this material and most likely resulting in a high-profile journal publication.
[1] external page K. Povarov et al., Phys. Rev. Research 2, 043384 (2020)
[2] external page L. Facheris et al., Phys. Rev. Lett. 129, 087201 (2022)

Published 01/11/2022
Contact: or
Quantum spin liquids (QSLs) [1,2] are novel collective quantum spin states where long-range magnetic order has been suppressed by quantum fluctuations. Theorists have predicted that QSLs exhibit many interesting properties, such as fractionalized spin excitations. This may allow quantum mechanical encryption and transportation of information that can be protected against environmental influences. Experimentalists have devoted great efforts searching for QSL states in several different candidate materials, mostly geometrically frustrated quantum magnets. However, solid, unambiguous evidence for these exotic states has not been found yet.
We have recently grown several new QSL candidate materials based on triangular lattice quantum magnets. In this project, you will perform thermodynamic and magnetic measurements on these materials at ultra-low temperatures and high magnetic field, to characterize their ground state and potential magnetic-field-induced phase transitions. The ultimate goal is to look for experimental signatures of a QSL state.
[1] external page L. Balents, Nature 464, 199 (2010).
[2] external page L. Savary and L. Balents, Rep. Prog. Phys. 80, 016502 (2017).
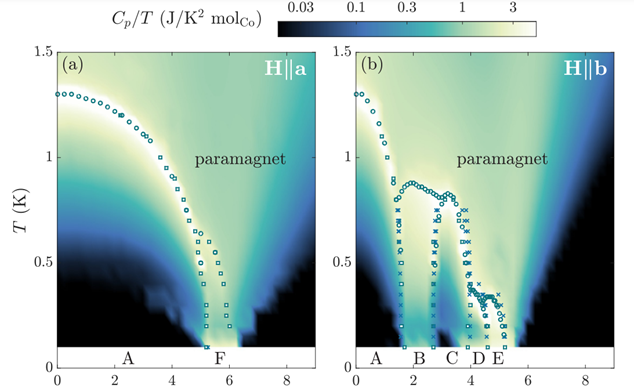
Published 01/11/2022
Contact: or
Spin-density wave (SDW) states are the epitome of quantum magnetism. Semiclassical models of spin insulators forbid their existence. More sophisticated quantum field theories predict their realization in particular triangular lattice systems, where coupling antiferromagnetic chains in a frustrated zig-zag manner boosts longitudinal correlations leading to the SDW order.
We have found the world’s first experimental realization of a 2D SDW in Cs2CoBr4 at low-field [1,2]. Surprisingly, one of the high-field “pre-saturation” states bears scattering signatures of possibly another SDW state. Its peculiarity is the extreme robustness: it appears to exist for any orientation of the magnetic field.
Clarifying this observation is the goal of this semester project. You will perform angle-dependent thermodynamic measurements at ultra-low temperature and high magnetic field. This will give us insight into the stability of the phase diagram. Combining these data with our scattering results will clarify the origin of this presaturation state and its angle-dependency.
[1] external page K. Yu. Povarov, et al., Phys. Rev. Research 2, 043384 (2020)
[2] external page L. Facheris, et al., Phys. Rev. Lett. 129, 087201 (2022)
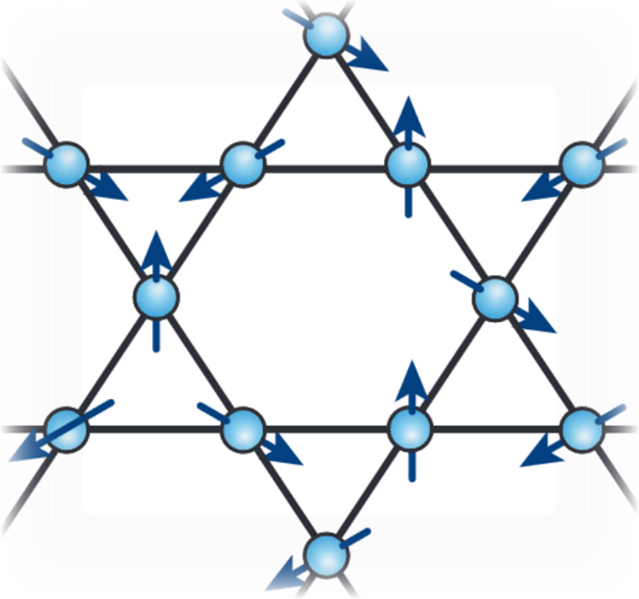
Published 01/11/2022
Contact: or
Geometric frustration remains one of the key topics in quantum magnetism, as it typically inhibits classical long-range order and instead promotes exotic quantum phases of matter. Perhaps the most archetypical of models to study magnetic frustration is the S = 1/2 kagome antiferromagnet, realizing a corner sharing lattice of triangles. According to long-standing theoretical predictions, it should realize the highly sought-after quantum spin liquid ground state [1], which is characterized by long ranged entanglement between spins without any spontaneous symmetry breaking, as well as fractionalized excitations. However, the hunt for experimentally suitable model materials is still ongoing, as most candidates studied so far are plagued by effects of structural disorder or additional terms in the Hamiltonian. Therefore, the recently discovered disorder-free family of rare-earth based kagome lattice antiferromagnets RE3BWO9 [2] holds tremendous promise. Several species show no signs of ordering down to the lowest temperatures, making them prime candidates to study spin liquid physics.
In this Semester project, you will investigate the thermodynamic properties in a single member of the RE3BWO9 family of frustrated quantum magnets. You will learn state-of-the-art techniques of performing calorimetric and magnetic measurements at ultra-low temperatures (down to 50mK) and high magnetic fields (up to 14 T). This will allow you to map out the entire phase diagram, marking an important step in the study of these exciting new quantum materials. The results obtained during this study are expected to be an essential contribution to a high-profile journal publication.
[1] external page L. Balents, Nature 464, 199–208 (2010)
[2] external page M. Ashtar et al., Inorg. Chem. 59, 5368 (2020)
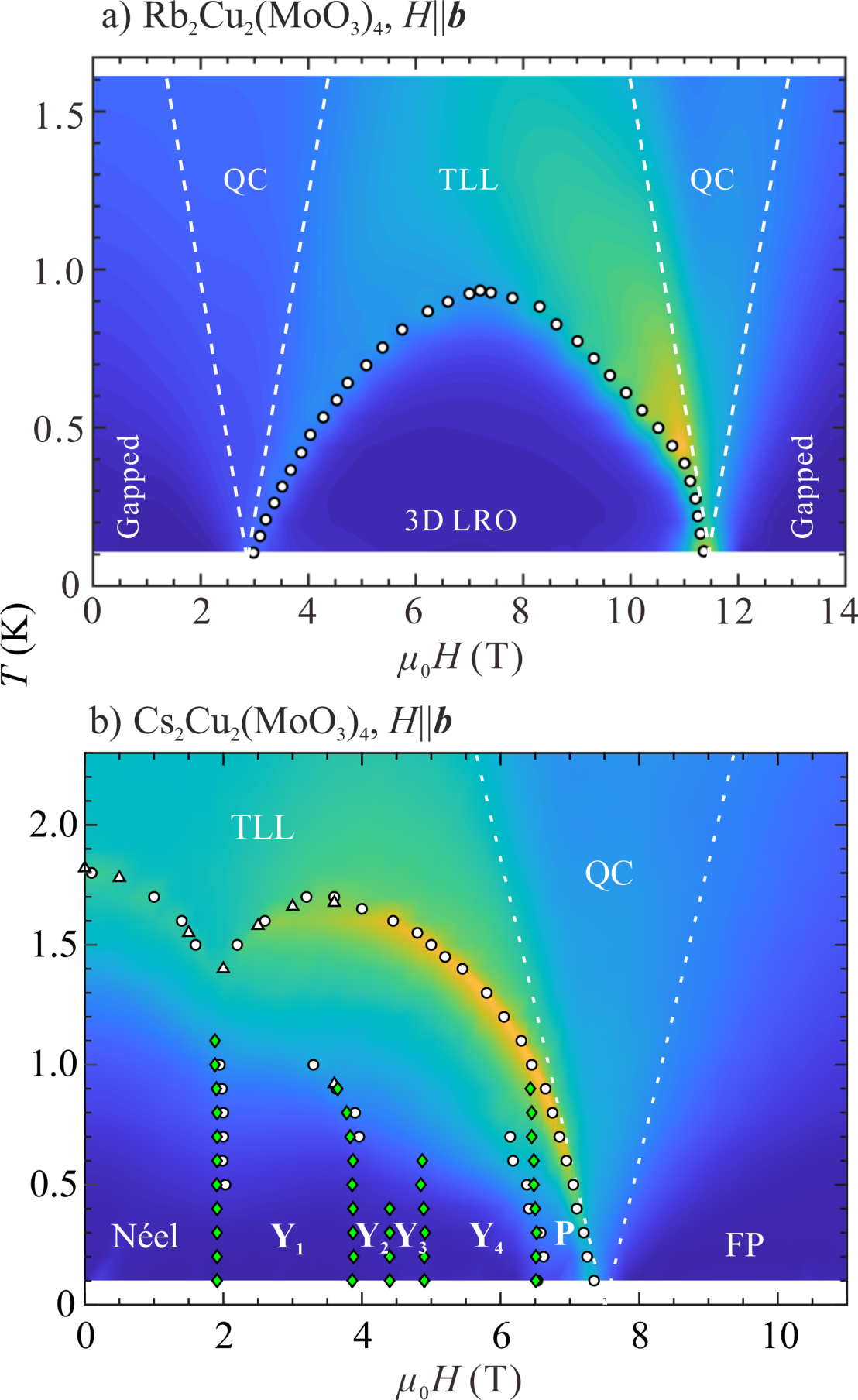
COMPLETED: No longer available
Contact: or
One dimensional magnetic systems are a fantastic playground for the study of quantum phases of matter. Adding competing interactions results in a zoo of unusual quantum phases, since any classical order is often washed out. In this regard, the spin chain molybdates A2Cu2(MoO3)4 (with A = Rb or Cs) are specially interesting. Despite their similar crystal structure, the subtle interplay between exchange interactions results in very different magnetic ground states. In the Rb-based compound a quantum paramagnetic ground state has been reported [1] and very peculiar dielectric properties have been found. The Cs-counterpart realizes an antiferromagnetic ground state where a handful of phases have been observed under magnetic fields, including an intriguing presaturation phase for which spin-multipolar condensates (such as spin-nematics) have been predicted [2].
In this Masters Project you will dive into the characterization of the spin excitation spectrum in these systems. For that you will get involved in inelastic neutron scattering experiments at NIST or Oak Ridge in USA. Then, you will deduce an effective spin Hamiltonian for these materials based on a thorough analysis of the data. This study will provide insight on the intimate differences between these magnetic systems. It will unveil the complex interactions taking place in the presumed simple Rb2Cu2(MoO3)4 spin chain and certainly draw conclusions about the realization of spin-nematicity in the Cs system. This project will also most probably contribute to the publication of a paper in a high impact journal.
References:
[1] external page S. Hayashida et al., Phys. Rev. B, 100, 134427 (2019).
[2] external page D. Flavian et al., Phys. Rev. B 101, 224408 (2020).
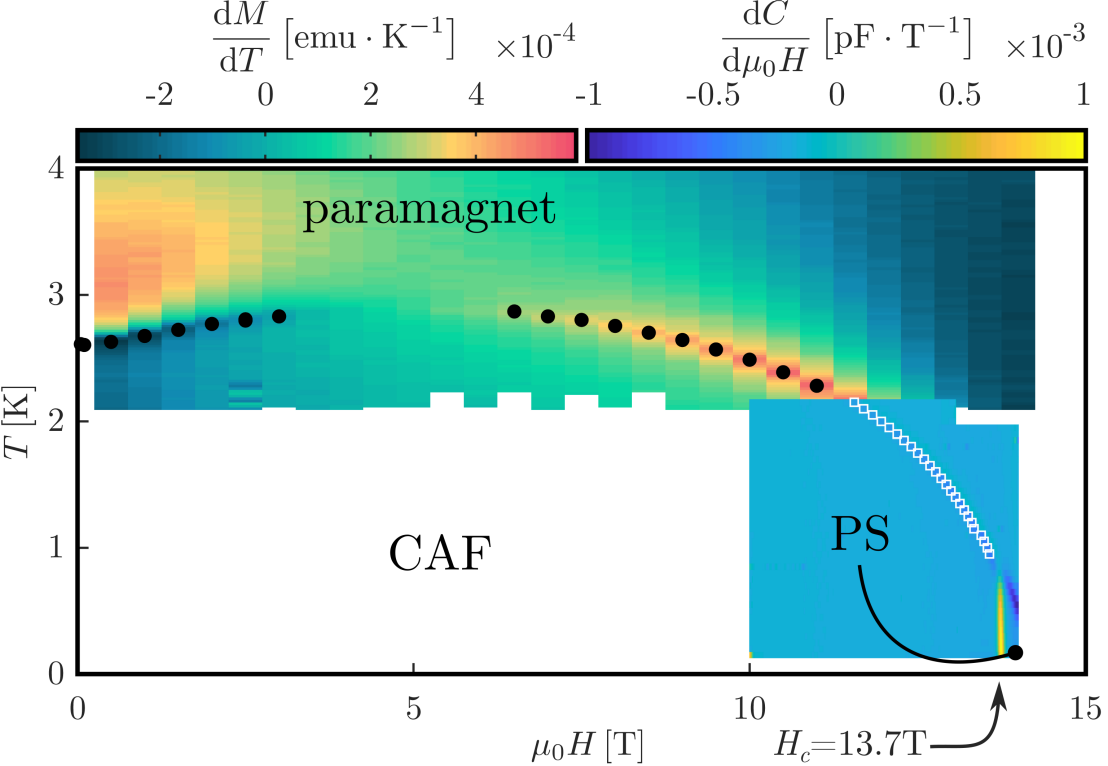
COMPLETED: No longer available
Contact: or
Classical magnets with competing ferromagnetic and antiferromagnetic interactions may switch from one type of magnetic structure to the other depending on their relative strength. In quantum magnets such a direct ferro-antiferro transition is prevented by zero-point spin fluctuations. Instead, a novel and often very exotic quantum phase must appear between the two classical states. The effect is enhanced in strong magnetic fields[1]. The most sought-after state of this kind is the so-called quantum spin-nematic[2].
In this Masters Project you will investigate a recently discovered pre-saturation phase in the frustrated quantum ferro-antiferromagnet SrZnVO(PO4)2. You will participate in a week-long high magnetic field inelastic neutron scattering experiment in Grenoble, France. You will then perform a very sophisticated analysis of the collected data in order to determine the magnetic excitation spectrum. This study will most certainly clarify the nature of the new high-field phase and hopefully prove it to be a quantum spin nematic, a first for this class of systems. The project has a high chance of success and will likely result in a nice paper in a high profile Physics journal.
[1] H. T. Ueda & T. Momoi, external page Physical Review B, 87, 144417, (2013).
[2] Schmidt, B. & Thalmeier, P., external page Elsevier Phys. Rep., 703, 1-59, (2017).
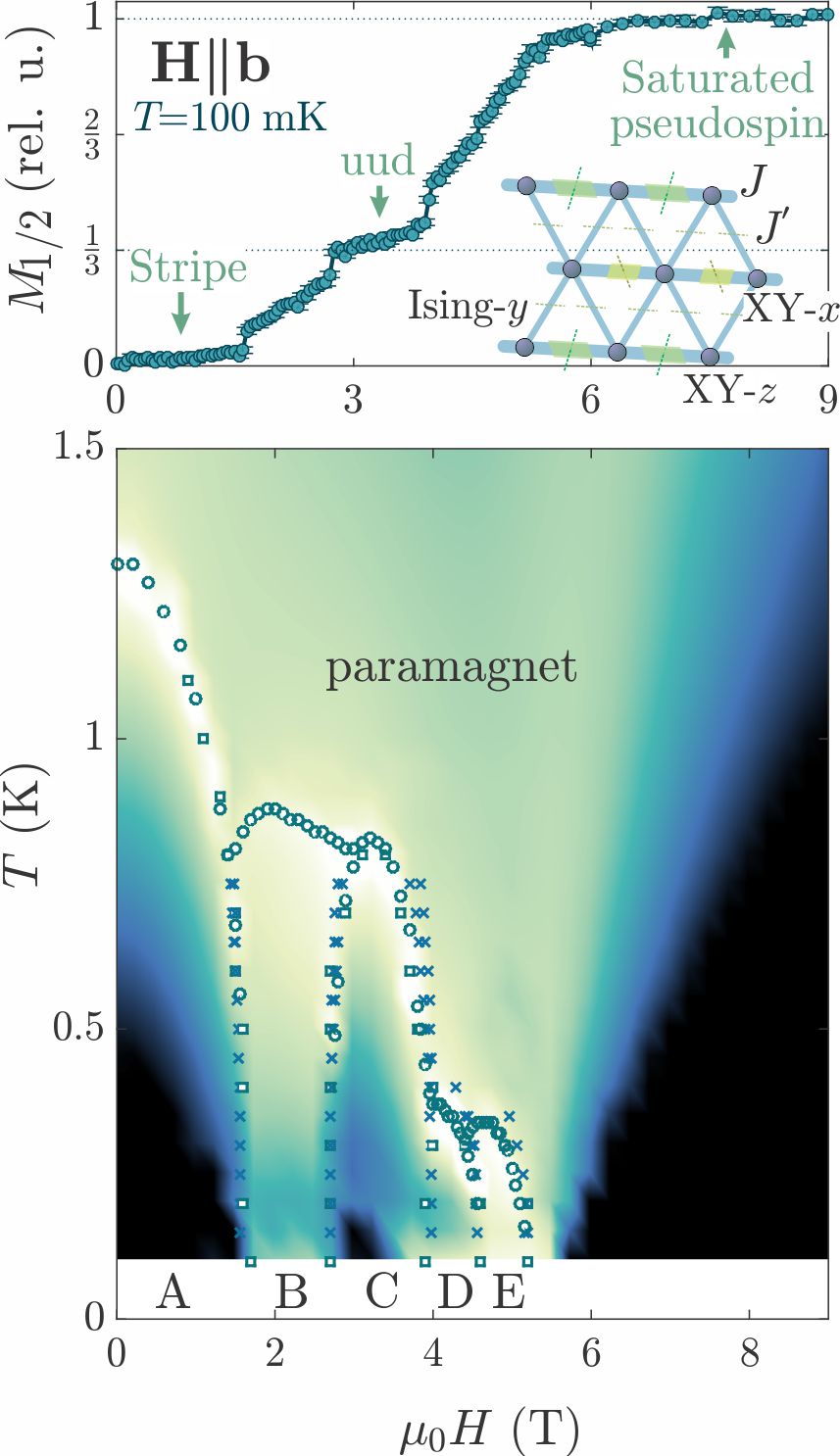
COMPLETED: No longer available
Contact: or
In the constant hunt for new exotic spin states, lattice frustration is often the starting point. Anisotropy, if present, may compete with geometric frustration, eventually leading to unusual magnetic states (see Ref. [1] for an overview). This is exactly the case of Cs2CoBr4, a brand-new distorted triangular lattice material with S = 3/2 and strong easy-plane anisotropy.
From a preliminary analysis, it turned out that the anisotropy essentially reduces the problem to S = 1/2 effective degrees of freedom. In addition, its axis alternates between the sites, leading to direction-dependent interactions. The consequent magnetic Hamiltonian shows a rather complex phase diagram, which we already mapped out in our labs at ETH (see picture and Ref. [2] for the whole story). We reliably identified 5 magnetically ordered phases along the preferential crystallographic axis b, with 3 of them featuring magnetization plateaux. However, thermodynamics alone could not tell us much more.
Unveiling the magnetic structure in each phase is the goal of the current master’s project: you will perform a cutting-edge neutron diffraction experiment at ILL (Grenoble), aimed at determining the propagation vector for each state. You will also learn how to process neutron data to reconstruct how spins arrange in each phase. This would allow us to get insights into the complicated magnetic behavior of the material. We expect this study to essentially contribute to a high-profile journal publication.
[1] O. A. Starykh, external page Rep. Prog. Phys. 78, 052502 (2015)
[2] K. Yu. Povarov, L. Facheris, S. Velja, Z. Yan, S. Gvasaliya and A. Zheludev,
external page arXiv:2004.09893 (2020).
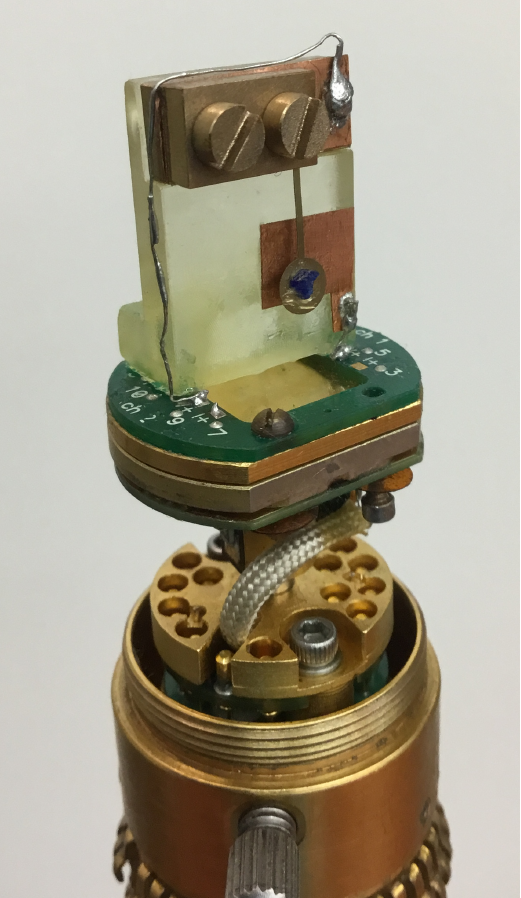
COMPLETED: No longer available
Contact:
Magnetism is an important player in many of the exciting topics in condensed matter physics: just think of unconventional superconductivity or exotic quantum states in magnetic insulators to name only two fields of current research.
Naturally, measuring uniform magnetization is one of the first steps in any experimental study of a material where magnetism is relevant. Down to a few Kelvin there are several techniques routinely used to obtain precise measurements of magnetization. However, at temperatures below 2 Kelvin, measuring magnetization becomes very difficult.
In our group we have already built a ‘proof-of-concept’ Faraday-force-magnetometer that will enable measurements of magnetization down to 0.05 Kelvin [1]. The present project encompasses the development, testing and commissioning of a reliable experimental setup for magnetization measurements at these ultra-low temperatures and in high magnetic fields.
Once working, this setup will be one of only a few in the world and we have samples of several interesting materials where measurements of magnetization at these low temperatures would be extremely valuable, in particular for the study of quantum phase transitions, i.e.
zero-temperature transitions between different ground states [e.g. 2,3].
A student working on this project will participate in all aspects of experimental condensed matter physics. First you will learn skills in designing and building measurement equipment and ultra-low temperature experiments. Then you will make use of the new measurement setup to investigate magnetization near quantum critical points in magnetic insulators featuring quantum phase transitions.
[1] for a description of a Faraday magnetometer see e.g.
T. Sakakibara, et al. external page Jpn. J. Appl. Phys. 33 5067-5072 (1994).
[2] K. Yu. Povarov, et al. external page Phys. Rev. B 96, 140414(R) (2017).
[3] K. Yu. Povarov, V. K. Bhartiya, Z. Yan, A. Zheludev, external page arXiv:1807.09549 (2018).
[4] Y. Feng, Master Thesis, ETH Zürich (2016).
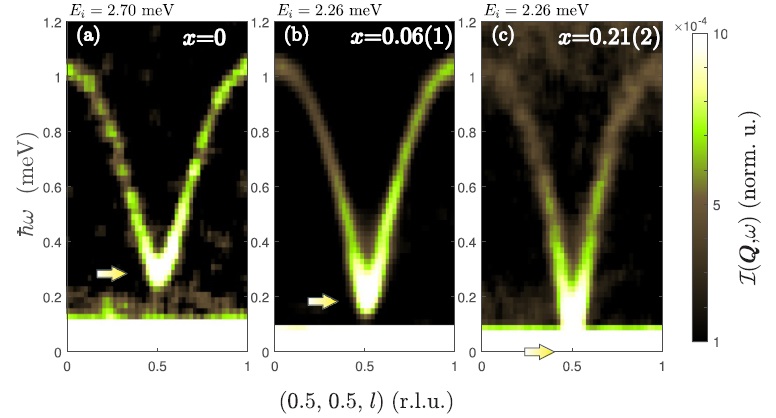
COMPLETED: No longer available
Contact:
The compound NiCl2.4SC(NH2)2 (a.k.a DTN) is one of the very frequently studied magnetic insulators. It contains Ni2+ ions which carry a spin 1. These spins then interact in a very well defined and ‘simple’ manner making DTN an excellent model system in the field of quantum magnetism.
Initially DTN became famous for a magnetic field induced antiferromagnetic phase which has been understood as a Bose-Einstein condensate of magnons [1]. More recently, Ni(Cl(1-x)Brx)2.4SC(NH2)2 ‘DTNX’ samples were synthesized with some of the chlorine atoms substituted by bromine. This strongly alters the interaction between the Spin 1 moments. Thus it opens the possibility to tune the spin interaction by varying the bromine content in DTNX [2]. At the same time, this opens the question how the introduced disorder affects the magnetism. Here, there is the tantalizing possibility of experimentally studying a Bose glass state, an exotic quantum state of a bosons in the presence of a random potential [3].
In our group we have succeeded in growing large high quality single crystals of both pure and bromine substituted DTN. This opens the possibility of a variety of exciting experiments such as: Inelastic neutron scattering experiments to investigate a quantum phase transition occurring at approximately 15% bromine substitution; or measurements of specific heat or magnetization at high magnetic fields and very low temperature to investigate possible bose glass physics.
As you can see, although long studied, there is a lot to be learnt from this compound and exotic physics to be explored. Moreover high quality samples are ready and waiting to be studied! This project can be easily adjusted in scope and will make an excellent semester or master’s project and might even grow into an interesting Ph. D. study in the field of quantum magnetism.
[1] V. Zapf, M. Jaime, and C. D. Batista. external page Rev. Mod. Phys. 86, 563 (2014).
[2] K. Yu. Povarov, et al. external page Phys. Rev. B 96, 140414(R) (2017).
[3] M. Dupont, et al. external page Phys. Rev. B 96, 024442 (2017).
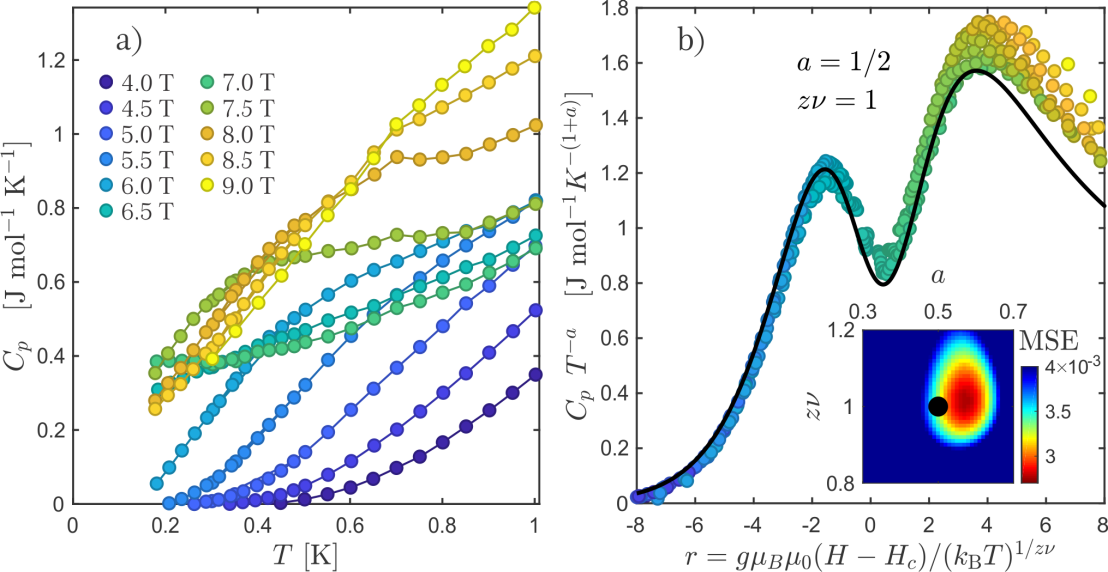
COMPLETED: No longer available
Contact:
Quantum critical points (QCPs) occur at zero temperature as a function of some non-thermal parameter when a system’s ground state abruptly changes. At first glance this seems like a rather abstract situation and certainly not relevant experimentally. However, it turns out, that the presence of a QCT can strongly affect physical properties in a wide parameter range and in particular at elevated temperatures [1].
Magnetic insulators offer particularly suitable model systems for the experimental study of such phase transitions. A prominent example are magnetic field induced transitions from a non-magnetic state at low fields to a magnetized state at high field.
Previously we have investigated such transitions in the quasi-one-dimensional spin ladder compound (C5H12N)2CuBr4 [2]. We have observed striking scaling behavior of specific heat data (figure). By means of inelastic neutron scattering experiments we have also in detail studied the critical dynamics near the transition.
In the present project we plan to investigate the compound (C5H6N2F)2CuCl4 [3]. This compound exhibits precisely the same magnetic field induced quantum phase transition. However, it has a layered structure and offers the possibility to study this QCP in two dimensions. This makes a huge difference as d=2 corresponds to the upper critical dimension for this particular transition and experimentally we are entering unchartered territory.
A student working on this project will perform measurements of specific heat and magnetization at low temperatures (down to 50 mK) and high magnetic fields (up to 14 Tesla) in our laboratory at ETH. To study the quantum critical dynamics you will take part in neutron scattering experiments performed at large scale neutron facilities. We expect this research to contribute to a publication in a peer-reviewed journal. Finally this project has the potential of growing into a nice PhD study in the field of quantum magnetism.
[1] S. Sachdev and B. Keimer, external page Physics Today 64, 29 (2011).
[2] D. Blosser et al. external page arXiv:1806.10392 (2018).
[3] T. Hong et al. external page Phys. Rev. B 83, 052401 (2011).
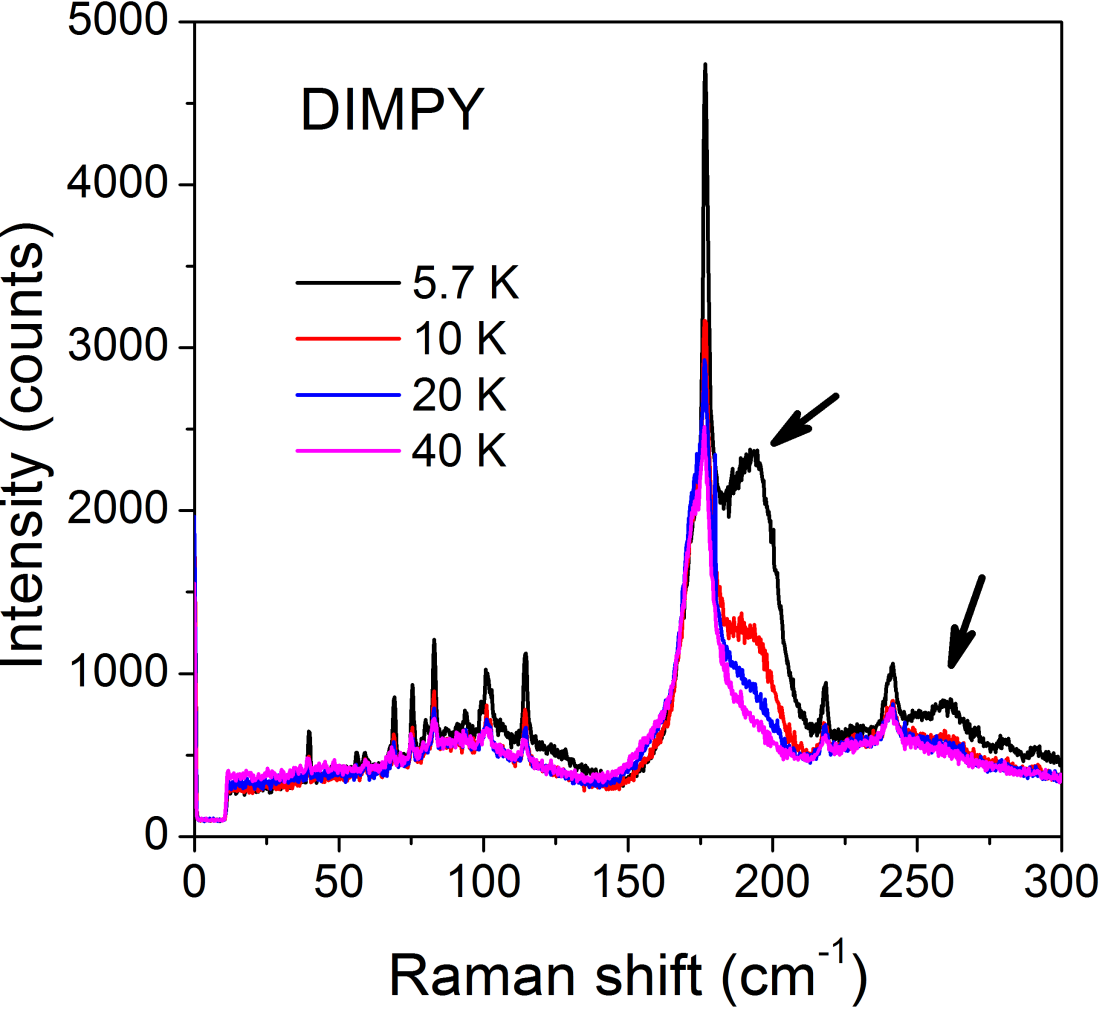
COMPLETED: No longer available
Contact:
Phonons are quasiparticles in solids, corresponding to collective excitations of the lattice. Similarly, magnons correspond to collective magnetic excitations. Usually, they interact weakly and can be treated separately. In some cases, however, they interact strongly resulting in more complex behavior. For example, in light spectroscopy experiments such effects would manifest in excitations, where magnons and phonons are created together forming a composite excitation.
We have performed preliminary light scattering measurements on a strong-leg quantum spin ladder (C7H10N)2CuBr4 [1] and have found indications of such excitations. This provides a unique opportunity to study magnon-phonon coupling in quantum magnets.
A student carrying out this project will perform Raman spectroscopy experiments on (C7H10N)2CuBr4. The phonon spectrum will be distorted and the effect on the magnon-phonon excitation will be measured. A simple way to perturb the phonon spectrum is to replace H by D in the compound and see what effect it has on magnetism. Interesting parallels with isotope effect in superconductivity can be drawn here [2].
The project can be potentially extended into a Master's thesis by including an alternative way to perturb the lattice by using hydrostatic pressure. It is significantly more challenging experimentally, but allows studying gradual changes in the spectrum and hence, a more detailed understanding of the coupling mechanisms.
A student working part of the NSM group will learn experimental skills of X-ray diffraction and Raman spectroscopy, as well as data processing techniques. We expect the research to contribute to a high-profile journal publication.
external page [1] Schmidiger et al., Phys. Rev. Lett. 108, 167201 (2012)
external page [2] recent example – Drozdov et al., Nature 525, 73 (2015)
![Enlarged view: Magnetocapacitive effect in Sul-Cu2Cl4 at ultralow temperatures (left) and the corresponding phase diagram (right). Each phase diagram point comes from the capacitive lambda-anomaly. See Ref. [4] for more information on this particular study.](/education/semester-and-diploma-projects/_jcr_content/par/accordion/accordionitem_0/par/textimage/image.imageformat.text50percent.525926578.jpg)
COMPLETED: No longer available
Contact: or
Combining nontrivial magnetic and electric properties within one material has always been a tricky problem, as they require a completely different type of symmetry breaking. Nonetheless, as it has been discovered about ten years ago, certain types of magnetic ordering do also trigger the electric polarization [1]. Naturally, such magnetic ordering also manifests itself in the dielectric properties of the material. This opens an exciting new way to learn something about the magnetic properties of exotic quantum antiferromagnets by just looking at their dielectric susceptibility [2, 3]. Needless to say, 'looking at properties' in the context of quantum magnetism means a combination of ultralow-temperature environment and high static magnetic fields, which is available right here, in our lab at ETH Hönggerberg campus.
Some first steps on this way have already been done with a highly frustrated S=1/2 material Sul-Cu2Cl4 (see figure). The careful examination of magnetocapacitive effect in this compound allowed us to obtain a brilliant quality phase diagram [4]. However, we already have a number of other candidate materials, for which a rich interplay between the electric polarization and quantum magnetism is expected. The aim of this Master's project would be to pursue those effects by using the unique setup created in NSM lab at ETH. The results obtained during this study are expected to be an essential contribution to a high-profile journal publication.
external page [1] M. Mostovoy, Phys. Rev. Lett. 96, 067601 (2006)
external page [2] F. Schrettle, S Krohns, P. Lunkenheimer et al., Phys. Rev. B 87, 121105 (2013)
external page [3] J. W. Kim, S. Khim, S. H. Chun et al., Nature Commun. 5, 4419 (2014)
external page [4] K. Yu. Povarov, A. Reichert, E. Wulf, A. Zheludev, Phys. Rev. B 92, 140410(R) (2015)
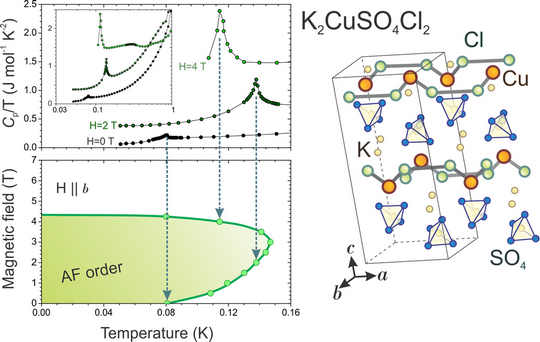
COMPLETED: No longer available
Contact:
"A good effective low-energy theory is worth all of quantum Monte-Carlo with Las Vegas thrown in," - once claimed famous P. W. Anderson [1]. This is especially true for the case of Luttinger Liquid (LL) theory, a one-dimensional analogue of Fermi Liquid paradigm [2]. The goal of the current research is the study of the phase diagram of slightly non-ideal 1D quantum system, a real spin-chain compound in our case (an example is shown in the figure below), which is expected to have some universal properties [3].
A student, working as a member of young and dynamic team, will learn state-of-art methods of dealing with ultra-low temperatures, high magnetic fields and calorimetric measurements under such a conditions. All the experiments will be conducted in NS&M Group laboratory at ETH Honngerberg campus. This perspective Semester project has all chances for turning into a more profound Master's project study, and we naturally expect it to contribute to a high-profile journal publication.
external page [1] P. W. Anderson and P. A. Casey, J. Phys.: Condens. Matter 22 164201 (2010)
[2] T. Giamarchi, "Quantum physics in one dimension", Clarendon Press (2004)
external page [3] H. J. Schulz, Phys. Rev. Lett. 77 2790 (1996)
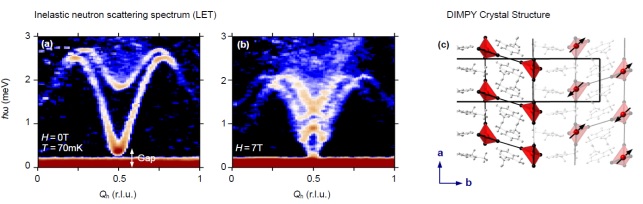
COMPLETED: No longer available
Contact:
Even though their ground states exhibit no long range order, low-dimensional quantum magnets reveal a cornucopia of both unexpected, exotic and theoretically very exciting physical phenomena. Among them, the Heisenberg S=1/2 spin ladder belongs to the most important and most studied model systems [1]. In zero-field, it remains in a complex many-body singlet ground state, a spin-liquid. The excitations with lowest-energy are sharp magnons carrying S = 1 and they exhibit a spin gap (fig 1a). In applied fields, these spin-liquids undergo a quantum phase transition to a novel exotic state which is nearly ordered, a Luttinger-Liquid. The sharp magnon excitations deconfine into a continuum of fractional S=1/2 spinon excitations and the gap vanishes (fig 1b).
The aim of the present work is to study the effect of site-disorder in a real Heisenberg spin-ladder. We focus on the recently discovered material (C7H10N)2CuBr4 (DIMPY, [2]) which was shown to be an almost perfect realization of the Heisenberg spin-ladder Hamiltonian [3,4]. In this material, the spin S=1/2 is due to the copper Cu2+ ions and site-disorder is introducted by random subsitution of Cu2+ with non-magnetic Zn2+. These 'missing spins' in the spin-ladder are predicted to affect the physics strongly. For example, low-energy degrees of freedom are released leading to localized states, 'spin islands' [5,6]. The phase diagram and thermodynamic response functions are hence strongly influenced by these low-energy quantum states.
We want to study these effects by careful magneto-thermodynamic studies. In our laboratory, we have grown a series of (C7H10N)2Cu1-xZnxBr4 crystals with 0<x<0.08. The aim of this work is to study the magnet-thermodynamic properties of Zn-disordered symples by specific heat and magnetization measurements at very low temperatures (down to 50 mK) and high magnetic fields (up to 14 Tesla) and to compare the results to measurements performed in the pure sample. As a Masters project, this project has many advantages. You will learn the use of cryogenic equipment and several commonly used experimental techniques.
This study is part of a larger project with various planned complementary experiments using different techniques both in- and outside our research group. It is hence likely, that the results of this thesis yield a nice publication in a peer-reviewed journal. Finally, this project has the potential of growing into a nice PhD study in the field of quantum magnetism, involving neutron spectroscopy (at our spectrometer at Paul Scherrer Institut), Raman spectroscopy and Muon Spin Rotation studies. Collaborations may include EPFL, Uni Geneva, Institut Laue Langevin andLaboratoire National des Champs Magnétiques Intenses (France) as well as Oak Ridge National Laboratory (USA).
external page [1] E. Dagotto, and T. M. Rice, Science 271, 618 (1996)
external page [2] A. Shapiro et al., Journal of the American Chemical Society 129, 952 (2007)
external page [3] D. Schmidiger et al., Phys. Rev. Lett. 108, 167201 (2012)
external page [4] D. Schmidiger et al.,Phys. Rev. Lett. 111, 107202 (2013)
external page [5] H.-J. Mikeska et al., Phys. Rev. B 55, 2955 (1997)
external page [6] A. Furusaki et al., Phys. Rev. B 52, 15930 (1995)