Light scattering
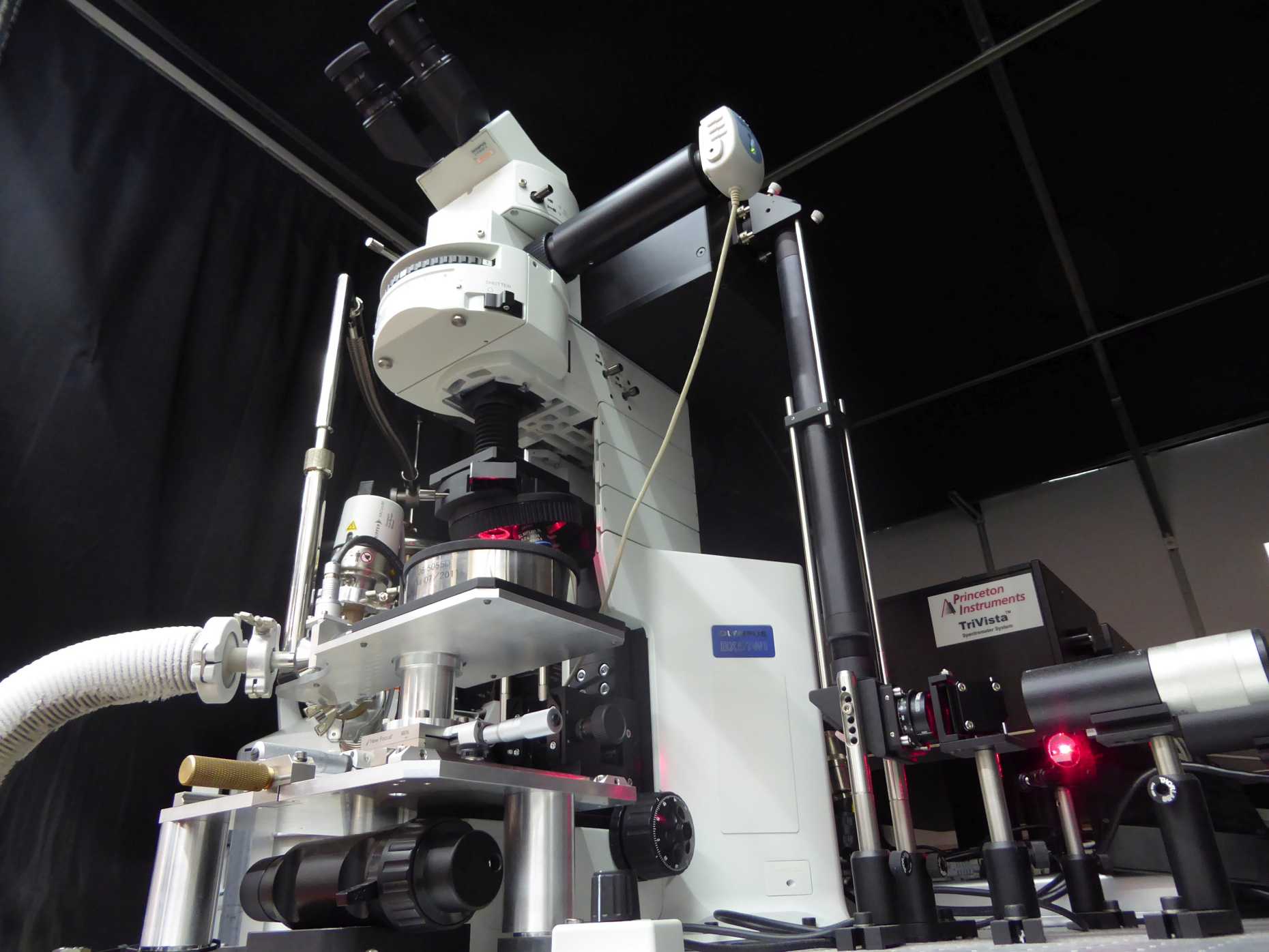
Light scattering is in many way complementary to neutron scattering. At the same energies relevant for the study of condensed matter systems light quanta have much smaller momenta than neutrons. Thus light scattering (Raman scattering), unlike neutron scattering, essentailly occurs at zero momentum transfer. The relative energy resolution that can be achieved in light scattering experiments is typically much better than for neutrons. Finally, light couples to lattice and magnetic degrees of freedom differently from neutrons, which means that it can see excitations which are invisible to neutron spectroscopy and electron spin resonance techniques.
For optical measurements we use a triple-grating high-resolution Raman spectrometer (S&I Spectroscopy & Imaging GmbH). The spectrometer is equipped with LN2 cooled back-illuminated CCD eXcelon camera detector to reduce noise. Experiments can be performed with the laser wavelenght 532 nm or 660 nm. The instrument is optimized for studying low-energy excitations and can collect data down to approximately 5 cm-1. It uses a continuous-He-flow cryostat to reach temperatures as low as 2.6 K.
Neutrons are S=1/2 particles. Angular-momentum conservation only allows the creation of a single S=1 magnon in a scattering event. In addition, singlet-to-singlet transitions are forbidden. In contrast, a photon is a S=1 particle. A typical Raman process creates pairs of magnons with opposing momenta, but single-magnon processes are also possible. Here is an example of a two-magnon excitation continuum (the broad feature at lower energies) measured in the strong-rung quantum spin ladder system Cu(Qnx)Br2. More details in external page G. Simutis, S. Gvasaliya, F. Xiao, C. P. Landee, and A. Zheludev, Phys. Rev. B 93, 094412 (2016).


An important advantage of light scattering is that it is very easy to perform experiments under high pressure, thanks to the use of (transparent !) diamond-anvil pressure cells. In out lab we can reach pressures up to 40 kbar, while still measuring at low temperatures down to 2.6 K. This technique was used to study the evolution of the magnon spectrum across a pressure induced quantum phase transition in the frustrated quantum dimer material (C4H12N2)Cu2Cl6. For more details see external page S. Bettler, G. Simutis, G. Perren, D. Blosser, S. Gvasaliya, and A. Zheludev, Phys. Rev. B 96, 174431 (2017).


Phonons are the easiest excitations to observe with Raman scattering. But are phonos of any use in the study of quantum magnetism? They most certainly are. Magnetic correlations between interacting spins contribute to the elastic energy and therefore stiffness of the corresponding bonds. This, in turn, affects the frequencies of phonons that involve distortions of the affected bonds. By measuring these anomalous phonon frequency shifts we can learn about spin correlations. This approach was used to study spin correlations in the famous material SrCu2(BO3)2 that realizes the Sutherland-Shastry frustrated dimer model. We study a paricular phonon exciation, the so-called "pantograph mode". We learn that, as the system goes through a pressure-driven phase transition from a dimerized to a quantum-plaquette phase, nearest-neighbor spin correlations switch from antiferromagnetic to ferromagnetic. For more details see external page S. Bettler, L. Stoppel, Z. Yan, S. Gvasaliya and A. Zheludev, Phys. Rev. Research 2, 012010(R) (2020).
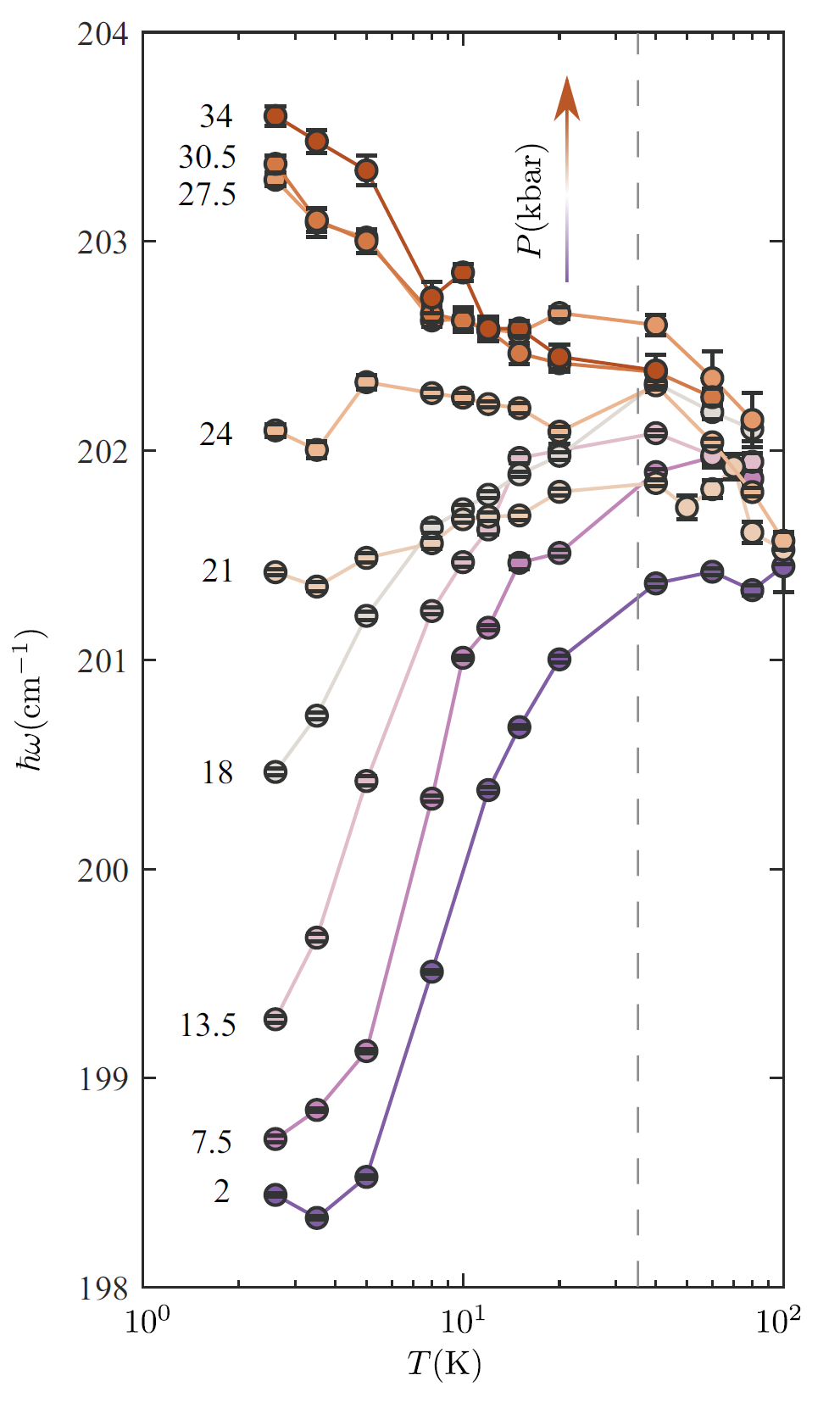